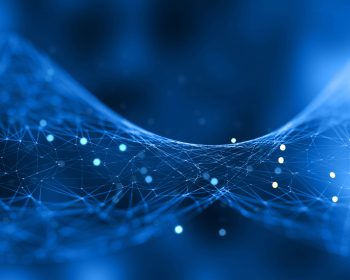
Written by: Khairul Haqeem, Journalist, AOPG.
As the world marches forward into a new era of technology, the potential for quantum computing looms large on the horizon. This revolutionary field of computing offers the promise of unprecedented processing power and speed, allowing for new solutions to complex problems and groundbreaking advancements in fields such as medicine, finance, and energy.
But as with any new field of study, there are countless hurdles to overcome before this potential can be fully realised. Enter quantum computing simulations: a critical tool in the quest to unlock the full potential of quantum computing.
Understanding Quantum Computing Simulations
In order to gain a comprehensive understanding of the capabilities of quantum computing simulations, it is imperative to possess knowledge regarding their fundamental principles and mechanics. Quantum computing simulations are essentially digital models that leverage the principles of quantum mechanics to replicate the behaviour of quantum systems. Complex structures such as molecules and materials pose a challenge to classical computing methods. As a result, these systems require alternative computational approaches.
Quantum computing research employs various simulation types, each possessing unique advantages and disadvantages. The Variational Quantum Eigensolver (VQE) is a simulation technique that aims to approximate the ground state energy of a given molecule or material. On the other hand, Quantum Phase Estimation (QPE) simulations are utilised to determine the eigenvalues of quantum systems. Additional simulation types encompass quantum Monte Carlo (QMC) and quantum annealing simulations.
Pioneering New Technologies With Quantum Computing Simulations
Quantum computing simulations have already been used to develop a range of new technologies with potential applications across a variety of industries.
Simulating quantum computers on a large-scale bears resemblance to high-energy physics simulations in that both require the management of a multitude of variables. The inputs and outputs of both are organised in a similar manner. In either scenario, it is necessary to examine and combine the simulations to produce outcomes.
As Dr Xioranny Linares, Quantum R&D and Business Director at Keysight Technologies notes, “Quantum computing offers a general framework for simulating physical quantum systems using several computational resources, that is polynomial in system size… These savings in computational cost can make a significant impact in diverse areas, such as drug discovery and material design.”
At Fermilab for instance, researchers are engaged in developing diverse applications for quantum computing simulations. These include high-energy physics data analysis, quantum theory and applications, large-scale simulations of quantum systems on high-performance computing, and quantum computation of fermion and boson systems. Researchers at Fermilab and other institutions are utilising simulations and physical experimentation to develop new technologies and investigate the potential of quantum computing to transform various industries and fields.
In particular, quantum computing simulations have the potential to revolutionise fields such as chemistry, physics, and materials science. By allowing researchers to model and test complex systems in a virtual environment, simulations can provide insights and solutions that would be difficult or impossible to achieve through physical experimentation alone.
Simulations vs. Physical Experimentation
Although quantum computing simulations provide various benefits, physical experimentation still holds significant value in quantum computing research. A significant benefit of conducting physical experiments is the direct observation and measurement of quantum systems’ behaviour, which can offer insights that simulations alone may not provide.
Notwithstanding, physical experimentation has its constraints. Due to the intricate nature of quantum systems, conducting experiments can be a costly and time-consuming process, posing a challenge for rapid iteration and testing of novel designs. Moreover, the potential of physical experimentation is constrained by the limitations of the experimental equipment, which may not be capable of comprehensively capturing the behaviour of intricate quantum systems.
In contrast to physical experimentation, simulations provide numerous benefits:
- Speed: Quantum computers possess the capability to execute specific categories of computations at a substantially faster pace compared to classical computers. The potential benefits of this are noteworthy in domains such as cryptography, astronomy, and financial modelling.
- Parallel Processing: The property of superposition enables a singular quantum computer to execute numerous calculations simultaneously. Because of this, quantum computers can do complicated computations at speeds much above those of conventional machines.
- Optimisation: Quantum computers excel in solving optimisation problems, which entail identifying the optimal solution from a vast array of possibilities.
- Simulation: The utilisation of quantum computers can facilitate the simulation of quantum systems that are challenging or unfeasible to simulate using conventional devices. This has significant ramifications for quantum chemistry and materials research.
- Cryptography: The potential of quantum computers to compromise prevailing encryption algorithms utilised for safeguarding sensitive information is a matter of concern. Certain encryption algorithms depend on the complexity of factoring large numbers, a task that can be readily accomplished by a quantum computer. Alternatively, quantum computers have the potential to facilitate the creation of novel and enhanced cryptographic techniques with heightened security measures.
Limitations of Simulations
With such benefits, quantum computing simulations offer immense potential for advancing innovative technologies. Nevertheless, there are still notable obstacles and constraints that must be addressed.
Jarrod McClean, a research scientist at Google’s Quantum Artificial Intelligence Lab, along with Alan Ho, a product manager at the same lab, and Shyue Ping Ong, an Associate Professor in the Department of NanoEngineering at the University of California, San Diego, in their research paper titled “The Promise and Challenges of Quantum Computing for Energy Storage“, said that the development of precise simulations for intricate quantum systems poses a significant challenge due to the restricted quantity of entangled qubits and fidelity available in contemporary quantum computers. The quantum operations that can be performed on the device are determined by this limitation. Although advancements are being made in the development of fault-tolerant quantum computers that can perform an infinite number of quantum operations, it may take several years for these machines to become available.
The lower limit for a quantum computer to be considered “useful” in simulating quantum chemistry systems is currently estimated to be approximately 128 orbitals, owing to the sophisticated nature of existing classical simulation techniques. In order to simulate complex compounds, it would have been necessary to perform over 10^12 gate layers approximately three years ago. State-of-the-art algorithms have significantly decreased these approximations to approximately 10^6. Nevertheless, there remains a considerable amount of progress to be made.
It is a challenging reality to accept that quantum computing simulations are constrained by resource limitations. Smaller organisations may encounter challenges when attempting to allocate resources towards the adoption of this technology, while researchers may face obstacles in conducting large-scale simulations.
A Future to Ponder Upon
The field of quantum computing simulations is rapidly evolving and holds immense potential to influence the future of computing. Quantum computers possess the capability to resolve computational problems that were previously deemed unsolvable, simulate physical quantum systems, and optimise machine learning problems. This breakthrough technology has the potential to revolutionise various industries and fields.
According to Dunlin Tan, who serves as the Director at Thales Research & Technology Singapore, it is imperative to maintain ongoing investments and research efforts in quantum computing simulations. This is essential to facilitate the creation of practical solutions and applications that can leverage the capabilities of quantum computers. Although the journey towards achieving fault-tolerant quantum computers may be lengthy, simulation platforms will emerge as a vital resource for researchers to expedite advancements and devise solutions that can have a positive impact on society. It is crucial to prioritise the cultivation of global quantum engineering proficiency and talent in order to advance this field.


Archive
- October 2024(19)
- September 2024(94)
- August 2024(100)
- July 2024(99)
- June 2024(126)
- May 2024(155)
- April 2024(123)
- March 2024(112)
- February 2024(109)
- January 2024(95)
- December 2023(56)
- November 2023(86)
- October 2023(97)
- September 2023(89)
- August 2023(101)
- July 2023(104)
- June 2023(113)
- May 2023(103)
- April 2023(93)
- March 2023(129)
- February 2023(77)
- January 2023(91)
- December 2022(90)
- November 2022(125)
- October 2022(117)
- September 2022(137)
- August 2022(119)
- July 2022(99)
- June 2022(128)
- May 2022(112)
- April 2022(108)
- March 2022(121)
- February 2022(93)
- January 2022(110)
- December 2021(92)
- November 2021(107)
- October 2021(101)
- September 2021(81)
- August 2021(74)
- July 2021(78)
- June 2021(92)
- May 2021(67)
- April 2021(79)
- March 2021(79)
- February 2021(58)
- January 2021(55)
- December 2020(56)
- November 2020(59)
- October 2020(78)
- September 2020(72)
- August 2020(64)
- July 2020(71)
- June 2020(74)
- May 2020(50)
- April 2020(71)
- March 2020(71)
- February 2020(58)
- January 2020(62)
- December 2019(57)
- November 2019(64)
- October 2019(25)
- September 2019(24)
- August 2019(14)
- July 2019(23)
- June 2019(54)
- May 2019(82)
- April 2019(76)
- March 2019(71)
- February 2019(67)
- January 2019(75)
- December 2018(44)
- November 2018(47)
- October 2018(74)
- September 2018(54)
- August 2018(61)
- July 2018(72)
- June 2018(62)
- May 2018(62)
- April 2018(73)
- March 2018(76)
- February 2018(8)
- January 2018(7)
- December 2017(6)
- November 2017(8)
- October 2017(3)
- September 2017(4)
- August 2017(4)
- July 2017(2)
- June 2017(5)
- May 2017(6)
- April 2017(11)
- March 2017(8)
- February 2017(16)
- January 2017(10)
- December 2016(12)
- November 2016(20)
- October 2016(7)
- September 2016(102)
- August 2016(168)
- July 2016(141)
- June 2016(149)
- May 2016(117)
- April 2016(59)
- March 2016(85)
- February 2016(153)
- December 2015(150)